Infrared light (IR) is an electromagnetic radiation whose wavelength spans between 780 nm and 1 mm, and it represents the less energetic, although the most abundant part (~54%) of the solar radiation spectrum that reaches the Earth’s ground (Fig. 1). It is an invisible radiation, and its main effect on human beings is the nice warmth sensation which most of us appreciate. However, since the beginning of the industrial revolution, in the 18th century, it is known that IR radiation can also produce dangerous consequences for the human eye: as the technological innovation was continuously providing new and stronger artificial sources of infrared light, studies were published associating the occupational exposure to IR with several kinds of eye injures, like retinal burns for welders, or cataracts, detected for the first time in glass blowers and metal workers already in 1739 [1].
The exposure to IR light has been related to several eye pathologies, mainly in association with thermal effects, acting on almost every part of the ocular anatomy [2] (Fig. 2); however, a particular care has to be taken when considering the contribution of IR radiation to the couple of diseases that most frequently are associated with aging: cataracts and Age-Related Macular Degeneration (ARMD). These two classes of injuries take place in different parts of the eye, respectively the lens and the retina. In both cases, the most relevant contribution to the formation of the damages is produced by IR-A, the energetically stronger part of IR, with wavelength spanning between 780 and 1400 nm.
Cataracts
Although the damaging effects of IR light on lens and the consequent formation of cataracts are known since more than a century, the understanding of IR actuation mechanisms has been largely debated. The first systematic study on fused glass workers was presented in 1886 [3]: a posterior cortical opacity was identified as a marking feature for the first stage of formation of IR-induced cataracts. In addition, during the second half of the 20th century the IR menace to human eye assumed a new and revolutionary form: as the laser technology became more available to industrial processes, the danger of injures for biological tissues increased [4]. Infrared lasers in particular, as they do not operate in the visible spectrum, do not emit light that can trigger a blink reflex.
A first attempt to explain IR-induced cataracts was published only in 1912 [5]: it was suggested that its formation could be related to the direct absorption of IR light by the lens, through a photochemical process.
Afterwards, a different theory achieved a larger acceptance: In the 30ies, Goldmann [6] suggested that the origin of cataracts was an indirect heating of the lens, induced by an IR absorption by the iris. This second hypothesis seems to be confirmed by more recent studies: several works of Södeberg et al. on mice’s eyes [7-9] demonstrated that a direct exposure to 1090 nm laser light cannot produce cataracts until the heating doesn’t reach a threshold temperature of 8 ºC, confirming that IRinduced cataracts take place through a thermal mechanism.
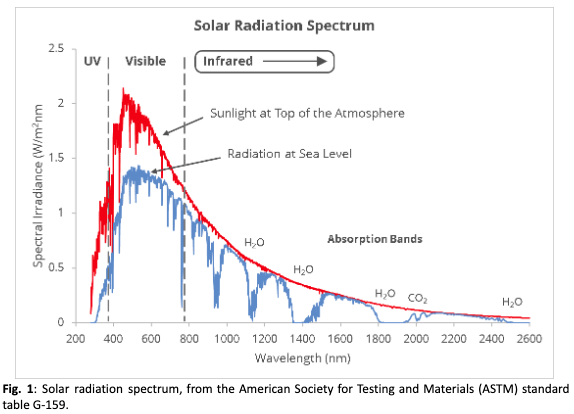
In the case of lasers, light emitted in the infrared portions of the electromagnetic spectrum, such as the CO2 laser, are again absorbed by the anterior portion of the eye, namely the cornea and lens which have a very high aqueous composition. It is this water that absorbs the laser energy resulting in thermal injury to the tissue. It is important to note that ocular injury can occur not only from direct exposure to the laser beam, but also from any light scattered off from reflective surfaces such as glass, polished metal, or plastic surfaces.
All the studies trying to establish the maximum level of IR radiation tolerable by the human eye, before cataracts appear, make use of high intensity sources [10]; however, it is worth pointing out that chronic exposure to a relatively low radiation intensity could lead to similar effects: a research presented in 1940 revealed how, during the first decades of the 20th century, the number of cataracts cases in Iowa used to increase significantly in the years following droughts [11]. Furthermore, mathematical models [12] suggest that, although occupational cataracts seem to be generated mainly by high intensity IR-B and IR-C sources (with wavelength respectively between 1400 and 3000 nm and between 3000 nm and 1 mm) through a heat transfer from the cornea to the lens, IR-A wavelengths are equally able to produce cataracts, following the mechanisms described by Goldmann. In addition, epidemiology data [13] at global scale evidenced how the highest percentages of visual impairment due to cataracts are found in the north African and south Asian regions: although the main reason has to be seen in the lack of accessibility to health care and limited financial resources for patients, it is interesting to observe how these areas are positioned at the equatorial latitude, where sun radiation is more intense.
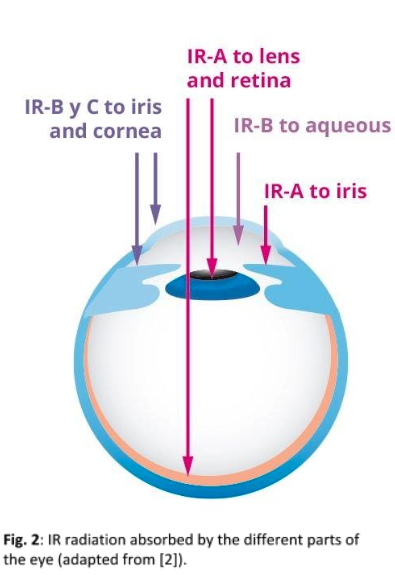
All the studies trying to establish the maximum level of IR radiation tolerable by the human eye, before cataracts appear, make use of high intensity sources [10]; however, it is worth pointing out that chronic exposure to a relatively low radiation intensity could lead to similar effects: a research presented in 1940 revealed how, during the first decades of the 20th century, the number of cataracts cases in Iowa used to increase significantly in the years following droughts [11]. Furthermore, mathematical models [12] suggest that, although occupational cataracts seem to be generated mainly by high intensity IR-B and IR-C sources (with wavelength respectively between 1400 and 3000 nm and between 3000 nm and 1 mm) through a heat transfer from the cornea to the lens, IR-A wavelengths are equally able to produce cataracts, following the mechanisms described by Goldmann. In addition, epidemiology data [13] at global scale evidenced how the highest percentages of visual impairment due to cataracts are found in the north African and south Asian regions: although the main reason has to be seen in the lack of accessibility to health care and limited financial resources for patients, it is interesting to observe how these areas are positioned at the equatorial latitude, where sun radiation is more intense.
Nowadays, it is commonly accepted that the main effect of IR radiation on the ocular lens is an accelerated aging [14], which produces cataracts. It is known that the aging process, involving oxidation events, is deeply affected by temperature and heat transfers. As a matter of fact, people who develop cataracts as a result of chronic or excessive exposure to IR radiation anticipate an opacification which was probably destined to appear in their old age years [15].
ARMD
The effect of IR exposure of the retina and the choroid is a temperature increase through the absorption of near-infrared energy by melanin (and, to a lesser extent, hemoglobin, see Fig. 3), which denatures enzymes. Generally speaking, temperatures only 10 ºC higher than the human body value could induce permanent thermal damages. In this field, enzymes called “matrix metalloproteinase” (MMP) have been largely investigated: this particular enzyme family plays an important role in maintaining and remodelling the tissues’ architecture. They can degrade every kind of extracellular matrix protein (among which we can find, for instance, collagen).
In 2003, a study presented by Lambert et al. [16] demonstrated that some type of MMP, namely the ones called MMP-2 and MMP-9, cooperate on the development of ARMD. Recently, Dadoukis et al. [17] were able to find a relationship between an increased activity of MMP-2, and consequently of ARMD, and chronic or severe exposure to IR radiation.
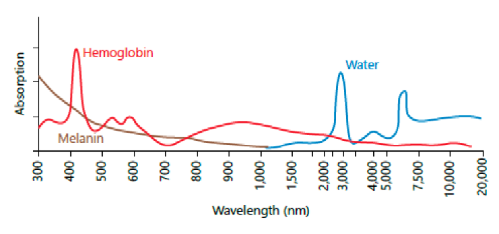
It is worth mentioning that, at the present time, MMPs have been found to act in virtually every tissue of the eye, both in health and disease condition [17, 18]: for instance, several groups demonstrated MMPs’ participation in the corneal surface reconstruction after a lesion, in the generation of corneal ulcers or of the dry eye disease. Moreover, a MMPs’ activity intensification in the lens has been related to cataractogenesis. Therefore, it is reasonable to believe that IR exposure acts in all these mechanisms through its relationship with MMPs.
Only recently, the scientific community showed an increasing attention toward the link between MMPs activity in the ocular tissues and the exposure to IR light, and many aspects of this topic still need to be disentangled. On the contrary, a large number of studies have already been performed about the IR reaction of human skin, and a certain parallelism can be found with the intra-ocular regions. It is commonly accepted that IRA radiation induces a general increase of MMP-1 activity [19, 20], which, in turn, can degrade collagen and other elastic fibers. Additionally, IR-A contributes to the creation of a free radicals’ excess [21], assuming a key role in the process of the skin photoaging: a chronic exposure to IR radiation can lead to changes in skin structure just like in the case of UV radiation. Nevertheless, there is not general concordance yet about the effective affectation of the human skin by solar infrared radiation: while the degradation mechanisms are generally accepted by the scientific community, the radiation intensity required to produce injures is still object of debate. Recent works point out that there is not compelling evidence to demonstrate that observable, deleterious cutaneous effects are occurring at doses of solar IR radiation corresponding to those experienced by populations in their normal environments [22]. Indeed, the exposition to relatively small intensities of IR-A could also have beneficial effects, since exposure to early morning IR-A wavelengths in sunlight may ready the skin for the coming mid-day deleterious UV [23].
Protection
Coming back to the eye, the idea of a prevalently deleterious effect is more consolidated, and a general consciousness about the need of eye protection versus UV light or occupational high intensity IR light is traditionally accepted. However, a consensus is growing about the idea that the effects of infrared radiation are cumulative throughout life, and a constant protection during the daily exposure is therefore desirable. Recommendations and legislations are already available concerning the protection requirements of sunglasses and safety eyewear [24-26], but with the increasing life expectancy, the diffusion of spare time activities in intense exposure environments (beach and mountain activities, sailing, etc.) and the growing presence in everyday life of artificial IR sources, like biometric, surveillance or heating devices, suggest that it is nowadays strongly convenient to incorporate the appropriate filters.
The engineering of an IR filter for ophthalmic lenses is a relatively novel question, which can be partly faced following the examples of other optical industries: the most experienced among them is the safety eyewear sector (producing the so called Personal Protective Equipment – PPE), where the protection strategy consists usually in incorporating IR absorbing dyes to the lenses, which can be on metallic or organic base: the nature and concentration of the tints depend on the use that the lenses are thought for, usually in combination with analogous UV absorbing pigments in PPE. This approach brings to an unavoidable consequence: all the available IR-A absorbing dyes are not transparent to visible light, so all the functional IR-A filters will appear dark at some extent.
Another example is the precision optics manufactory: here, bandpass elements centered in the visible region are needed, with a high transparency inside the nominal band, so they are built under a different approach. While the short wavelength region could be filtered again by absorbing dyes, the most common strategy for having a definite passing band, and in particular to eliminate the IR radiation, is to reflect out the unwanted wavelengths by an interference multilayer coating, opportunely tuned in thickness, number and composition of the layers, in order to obtain the sharpest possible frontier between the allowed and the blocked radiation.
In ophthalmic optics, the assembly of an effective IR filter is set on both the approaches previously mentioned: the issue is on the table since a relatively short time, but in the case of sunglasses, for instance, some degree of filtering was made available in the last decade by the inclusion of absorbing dyes, as for safety eyewear. However, for an effective concentration, the colorants must be included in the casted polymer of the semifinished lens (and not embedded on the finished product), and this almost excludes the applicability to prescription lenses.
An alternative can be inspired by the technology of heat mirrors [27], utilized in energy saving construction or solar energy production: here a thin layer of a metal, usually silver, is used for its low absorbance in the visible range, and high reflectance in the near IR at the same time [28]. However, the required Ag thickness and the need for protection from oxidation (usually by sandwiching the silver between nanolayers acting as barriers), contribute to reduce the total transmittance of the stack. The reduced transparency is not acceptable for a generic clear ophthalmic lens, so other approaches have been searched: several patents have been presented in the last years, showing that a quite relevant filtering action of IR-A (40-60%) can be obtained by a purely interferential effect in a multilayer ceramic stack, where high (>1.9) and low (<1.6) refractive index transparent materials are sequenced, just like in a standard anti-reflective coating. The efficiency of the filter increases with the number of the layers, and consequently with the total thickness of the stack [29]. However, a thick and very structured multilayer involves several mechanical and aesthetical issues, like the scratch and thermal resistance of the coating, or the filter efficiency and aspect at different angles, that should be avoided. The total thickness can be reduced combining a deep tuning of the layers’ composition with novel thickness solutions [30-32], diverging from the traditional repetition of a bilayer combination, utilized for instance in precision optics.
Nowadays, a functional filter for the near IR waveband is already available from several manufacturers for the full range of prescription lenses. Indeed, of course the radiation intensities that we are subjected to in our everyday life are not going to make us blind overnight, but evidences are clear: a cumulative effect is present, and it is potentially dangerous. So, if it’s not a question of running to put a filter on our lenses, let’s say it’ at least matter of fast walking.
References
[1] H.S. Turner, The Interaction of Infrared Radiation with the Eye: a Review of the Literature (Ohio State University Research Foundation, Columbus, Oh., 1972).
[2] J. Voke, Optometry Today 21, 22 (1999).
[3] W. Meyenhofer, Klin Monatsbl Augenh 24, 49 (1886).
[4] S. Bard, Laser Treatment of Vascular Lesions (Karger, Basel, 2014).
[5] A. Vogt, Arch. Ophthal. 83, 99 (1912).
[6] H. Goldmann, Arch. Ophthal. 9, 314 (1933).
[7] Z. Yu, et al., Journal of Biomedical Optics 20, 15003 (2015).
[8] Z. Yu, et al., Journal of Biomedical Optics 19, 105008 (2014).
[9] P.G. Söderberg, et al., Eye 30, 241 (2016).
[10] D. G. Pitts, and A. P. Cullen, Albrecht von Graefes Arch Kiln Ophthalmol 217, 285 (1981).
[11] P.W. Salit, Acta Ophthalmologica 18, 309 (2009).
[12] T. Okuno, Ann. Occup. Hyg. 38, 351 (1994).
[13] D. lam, et al., Nat. Rev. Dis Primer 1, 15014 (2015).
[14] E. Lydhal, and B. Philipson, Acta Ophthalmologica 62, 961 (1984).
[15] P.G. Söderberg, in Light, Lasers and Synchrotron Radiation: a Health Risk Assessment, edited by M. Gandolfo, A. Rindi, and D.H. Sliney (Plenum Press, New York, 1991), pp. 219–228.
[16] V. Lambert, et al., The FASEB Journal 17, 2290 (2003)
[17] P. Dadoukis, et al., Graefes Arch Clin Exp Ophthalmol 251, 1929 (2013).
[18] J. M. Sivak, and M. E. Fini, Progress in Retinal and Eye Research 21, 1 (2002).
[19] P. Schroeder, et al., Journal of Investigative Dermatology 128, 2491 (2008).
[20] S. M. Schieke, et al., Journal of Investigative Dermatology 119, 1323 (2002).
[21] L. Zastrow, et al., Hautarzt 60, 310 (2009).
[22] B. Diffey and B. Cadars, Photochem. Photobiol. Sci. 15, 361 (2016).
[23] D. Barolet, et al., Journal of Photochemistry and Photobiology B: Biology 155, 78 (2016).
[24] B. A. J. Clark, The Australian Journal of Optometry 52, 167 (1969).
[25] N. Kourkoumelis and M. Tzaphlidou, The Scientific World JOURNAL 11, 520 (2011).
[26] International Commission on Non-Ionizing Radiation Protection, Health Phys. 105, 74 (2013).
[27] J. C. C. Fan, and F. J. Bachner, Applied Optics 15, 1012 (1976).
[28] A. Carrilero, et al., Spanish Patent ES2354351A1 (2011).
[29] Y. Matsui, et al., Japanese Patent JPWO2015080160A1 (2013).
[30] F. Rigato, et al., Spanish Patent ES2536827B1 (2013).
[31] H. Takahashi, Japanese Patent JP2015148643A (2014).
[32] X. Ding, European patent EP3242150A1 (2016).
Do the progressive
Article publish in MAFO magazine edition 1-19.
Infrared radiation
Article publish in MAFO magazine edition 4-18.